Preserving the Natural Wonder of Devils’ Town
The DEMONITOR Project (Devils’ Town Erosion Monitoring) is a scientific research initiative focused on studying and protecting one of Serbia’s most unique natural landmarks—Devils’ Town. This breathtaking site, located near Prolom Spa, consists of nearly 200 towering rock formations, some reaching up to 15 meters in height. These pillars, shaped over thousands of years by erosion, wind, and water, are continuously changing—some are collapsing, others are forming, and the landscape is shifting.
Recent concerns over climate change, increasing tourism, and natural weathering have raised urgent questions:
- How fast is Devils’ Town eroding?
- What factors influence these changes?
- Can we predict future shifts?
- Are there ways to slow down the erosion and preserve the site for future generations?
Our Mission: Understanding and Protecting Devils’ Town
DEMONITOR is a three-year research project that tracks these changes in real-time. Unlike previous studies that relied on simple visual observations, this project introduces cutting-edge scientific methods to accurately measure, analyze, and predict erosion trends. The collected data will help conservation efforts and contribute to the site’s potential recognition as a UNESCO World Heritage Site.
How We Monitor Changes?
To understand how Devils’ Town is evolving, DEMONITOR applies a combination of advanced, non-invasive monitoring techniques, including:
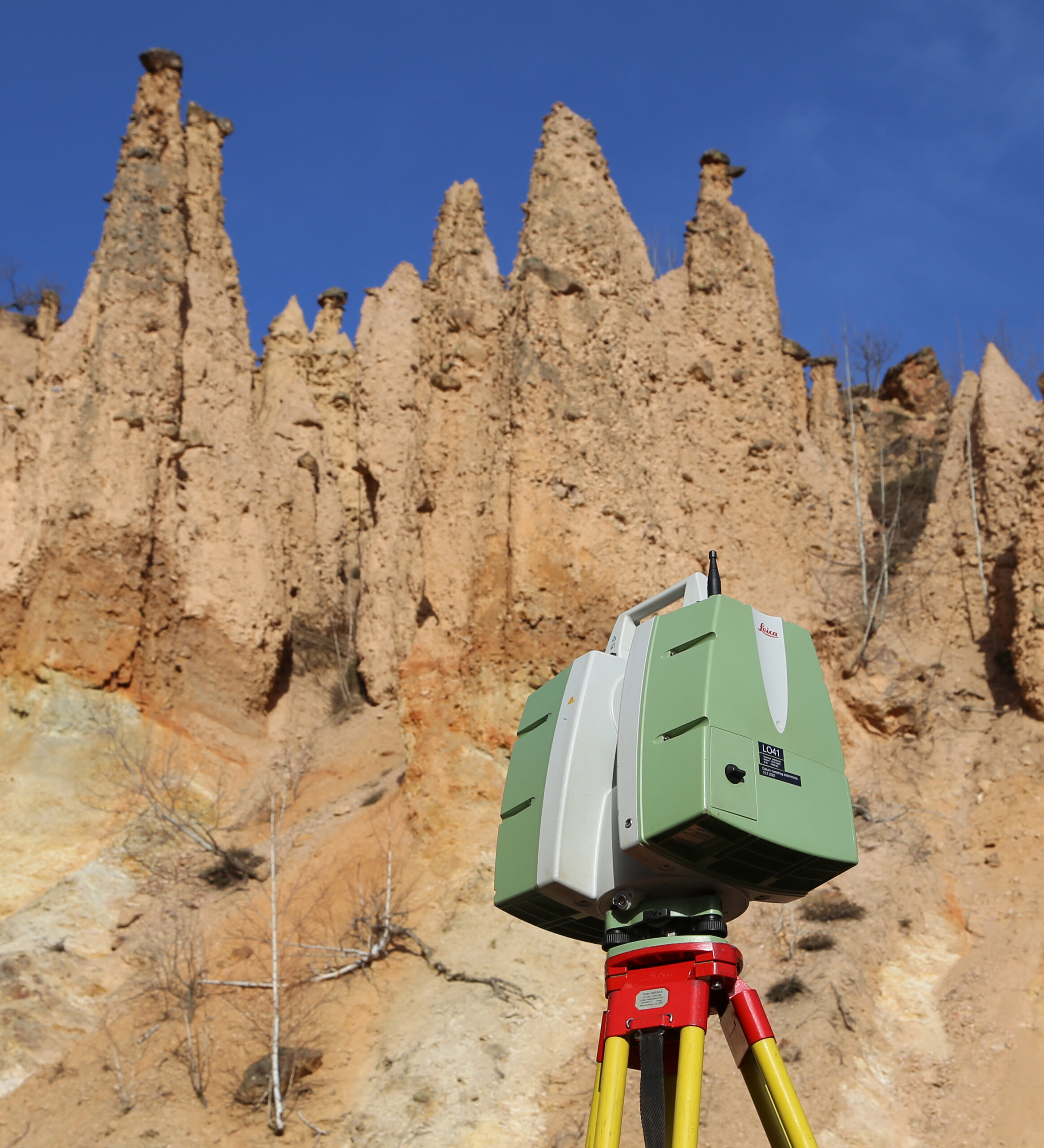
Terrestrial LiDAR (Laser Scanning) and drone Photogrammetry—These technologies create highly detailed 3D models of the rock pillars, allowing us to track even the most minor changes over time.
Satellite Radar Interferometry (InSAR): Using satellite data, we can detect ground movements that may indicate instability or future collapses (Image: ESA/ATG medialab).
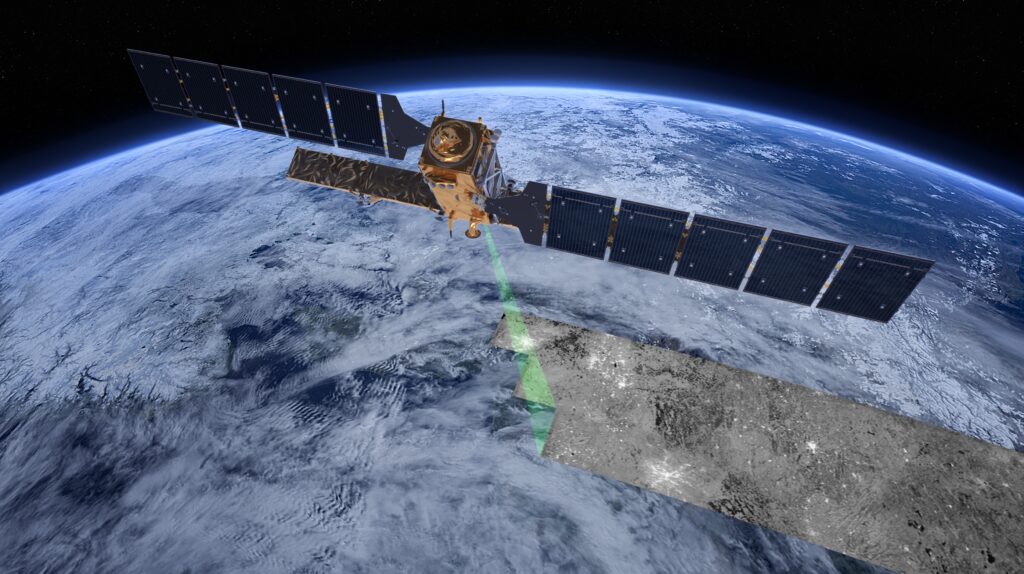
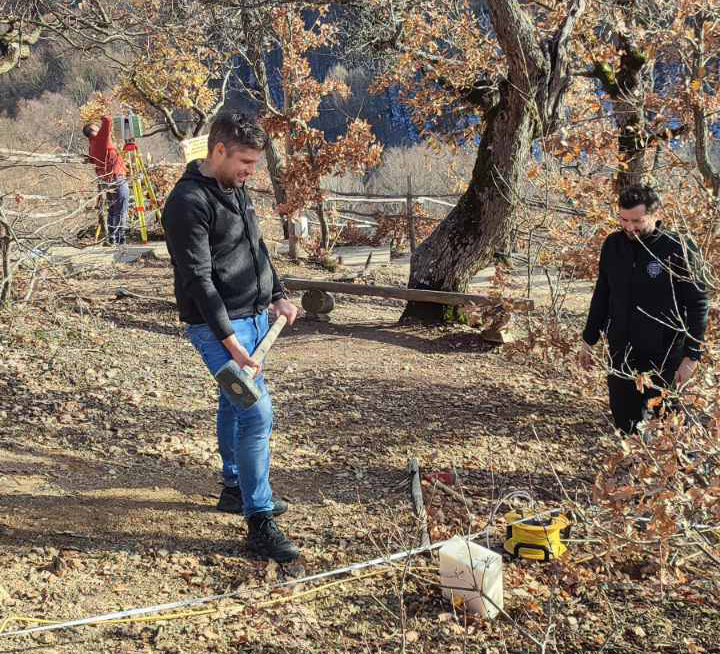
Seismic and Geoelectric Surveys – These methods help us study the subsurface conditions beneath the pillars, revealing weaknesses in the rock structure.
Time-Lapse Imaging & Weather Monitoring – A fixed camera system captures real-time images, allowing us to correlate erosion patterns with weather conditions, such as rainfall, temperature changes, and seasonal effects.
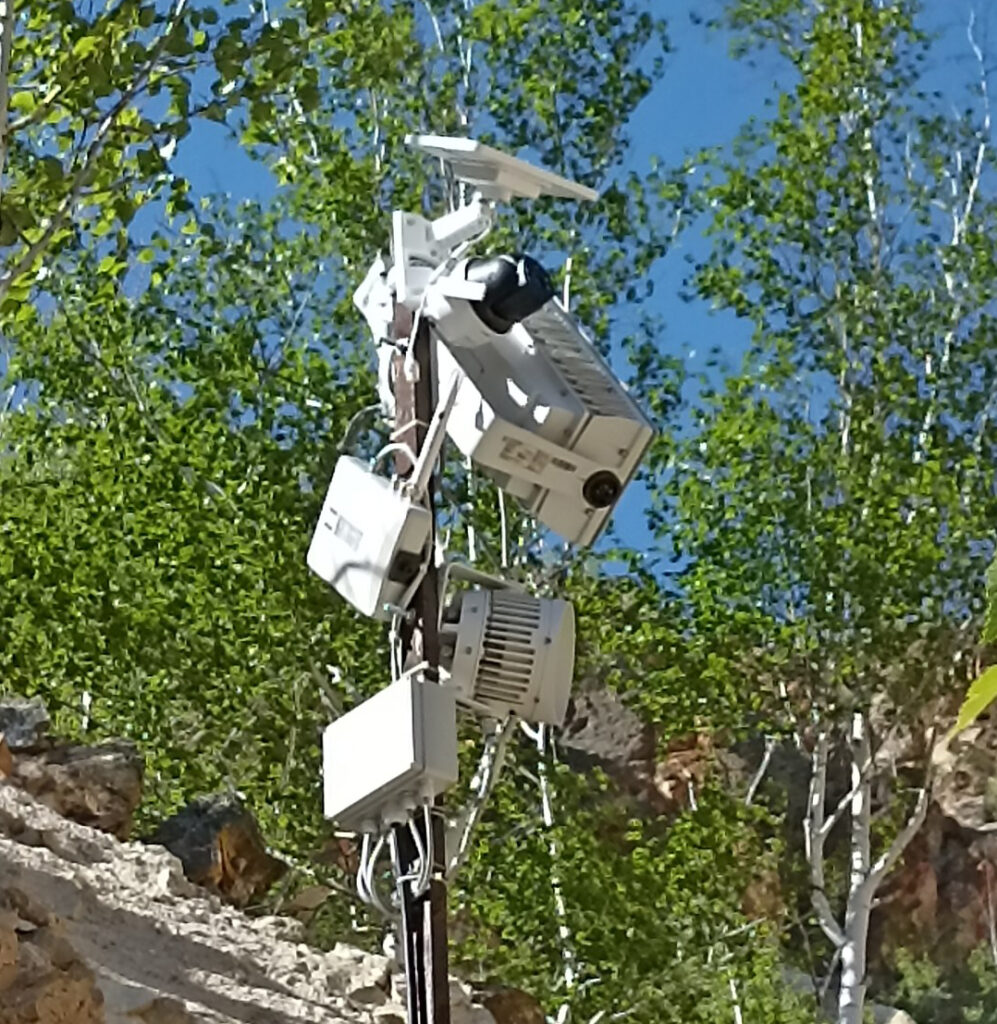
By combining these tools, the project will provide valuable insights into the erosion process and help predict how the landscape will evolve in the coming decades.
Why This Research Matters?
Scientific Impact
The DEMONITOR project’s findings will contribute to several important fields, including geomorphology, geology, geophysics, and climate science. Understanding how erosion shapes landscapes like Devil’s Town can help scientists study similar formations worldwide.
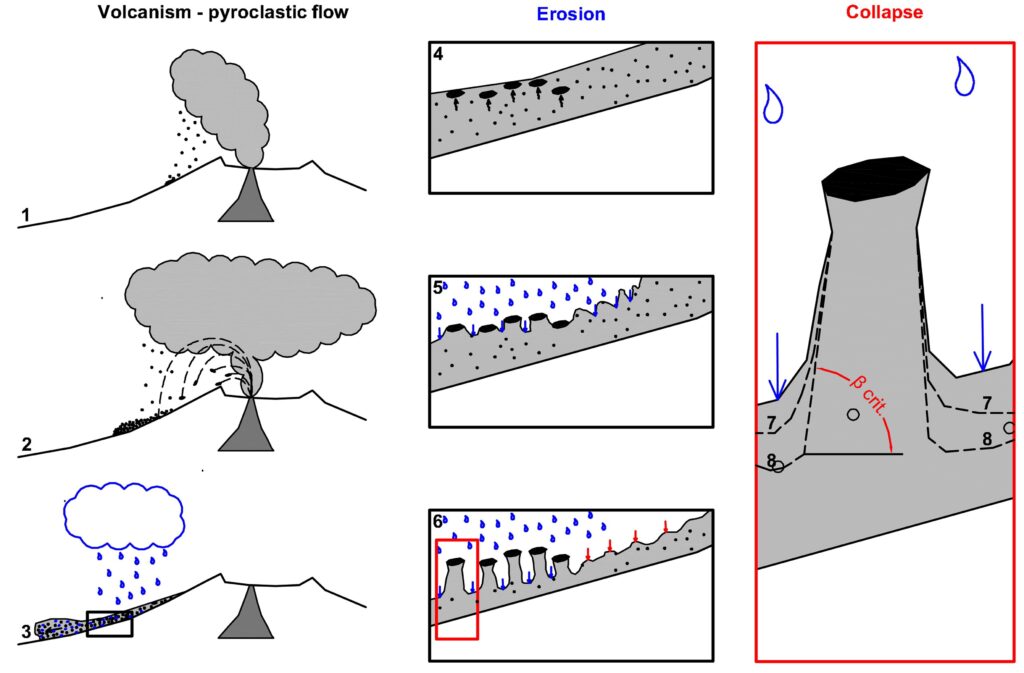
Conservation & UNESCO Recognition
Devil’s Town has been a protected natural monument on UNESCO’s tentative list for World Heritage status since 2002. However, securing full recognition requires more scientific data about the site’s uniqueness and significance. DEMONITOR will provide crucial evidence to support future UNESCO applications and international conservation efforts.
Tourism & Local Economy
The project also has a social and economic dimension. Devils’ Town is a major tourist attraction, and its continued preservation is essential for the local economy. By raising awareness about the site’s scientific and cultural value, we aim to
By combining science, conservation, and public awareness, DEMONITOR aims to preserve the magic of Devil’s Town for generations to come.
- Boost sustainable tourism, bringing more visitors while ensuring responsible site management.
- Provide educational resources, including 3D models and interactive content, for visitors to learn about the geological history of the site.
- Help local guides and conservation groups use scientific findings to create engaging stories and promote the site globally.
Looking Ahead: A Long-Term Vision
The DEMONITOR Project is just the beginning. Our ultimate goal is establishing a long-term monitoring program for Devils’ Town, ensuring continuous protection and data collection after the project ends. We also plan to share our methods and findings with researchers studying similar landscapes in North Macedonia, Turkey, and beyond.